Cracking the (Zip)code of Neural Cells
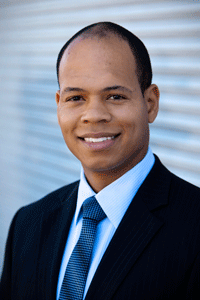
Randolph Ashton
Neural cells, derived from human pluripotent stem cells, have been used to successfully treat rodent models of epilepsy, Huntington’s, and Parkinson’s disease. These studies demonstrate the enormous potential of regenerative medicine to treat neurological disorders and traumatic injuries that damage our brain or spinal cord.
Administering synthetic neural cells to patients could replace cells that had been lost through disease or injury, for example. But in order to have the best regenerative effect when being used to treat neurological disorders or trauma to the central nervous system or CNS, introduced neural cells have to be specific to the anatomical region into which they are being transplanted. Until now, it has been frustratingly difficult for researchers to synthetically derive pure populations of neural cells characteristic of cells found at specific locations in the hindbrain and spinal cord.
In a paper published today in Stem Cell Reports, a team of researchers led by Randolph Ashton, Assistant Professor of Biomedical Engineering at UW–Madison, and a member of the BIONATES Theme at the Wisconsin Institute for Discovery, present a method that makes it possible to obtain highly homogenous populations of neural stem cells that have the potential to produce neural cells from any region of our hindbrain and spinal cord.
“We can now specify neural stem cells to different diverse, discrete regions throughout the hindbrain or spinal cord,” says Ashton, “and if we need to regenerate tissue that had been lost due to injury or disease, we can now customize neural stem cells and their neural cell derivatives for the particular location of transplantation,” he says.
In addition to helping us better research and treat neurodegenerative disorders such as spinal muscular atrophy (SMA) and amyotrophic lateral sclerosis (ALS), the ability to obtain large numbers of highly location-specific neural stem cells and neurons is expected to provide transformative insight into how our central nervous system, or CNS, is formed during embryonic development.
“When we normally derive neural stem cells, they are similar to the ones that give rise to the forebrain,” says Ashton. To generate tissues of the precise hindbrain or spinal cord locations, the challenge was to mimic precisely the complex patterns of which specific genes were turned on or off in neural stem cells found in these regions of our CNS.
“If you delete some of these [HOX] genes in a fruit fly you can get rid of entire body segments of the fly.”
–Randolph Ashton
Ashton and his colleagues had already devised a method to derive neural stem cells from human pluripotent stem cells in a way that could be used in a clinical setting. While trying to coax these neural stem cells to differentiate further into neural cells, such as motor neurons, they discovered the application of certain growth factors would cause these neural stem cells to turn on a particular set of genes called HOX genes.
Turns out, one of the key reasons why CNS tissue from your hindbrain is different from CNS tissue from half way down your spinal cord, for example, is that a different set of HOX genes are turned on or off, or expressed, depending on where neural stem cells are located in the CNS during embryonic development.
“It’s fascinating,” says Ashton, referring to the importance of HOX genes in the development of organisms from ranging from humans to fruit flies. “If you delete some of these [HOX] genes in a fruit fly you can get rid of entire body segments of the fly,” he says.
Studying the nuances of HOX genes expression patterns and levels of different HOX proteins in neural stem cells has implications in understanding not only the developmental processes that build the CNS, but also how different parts of our skin, skeletal muscles, limbs, and visceral organs are formed.
There are 39 HOX genes in the human genome, and which subset of these are expressed in neural stem cells depend on where in the CNS those cells are. “It’s useful to think of HOX genes as a zip code for neural stem cells,” says Ethan Lippmann, a post doctoral researcher in Ashton’s laboratory and lead author of the study. Depending on where in the hindbrain or spinal cord the neural stem cells end up, HOX genes are expressed in a pattern characteristic of that location.
Previous efforts had generated neural stem cells with mixed zip codes because researchers couldn’t precisely control how pluripotent stem cells differentiated into neural stem cells of the hindbrain or spinal cord. “But now we can specifically assign the neural stem cells to particular zip codes of the hindbrain or spinal cord reproducibly and predictably,” says Lippmann.
Ashton, Lippmann and the team used a cocktail of growth factors and signaling molecules to push human pluripotent stem cells along the path to becoming neural stem cells. These growth factors caused the pluripotent cells to start expressing HOX proteins in a manner that changes predictably over time.
The team discovered that by adding a chemical called retinoic acid at different times after HOX proteins had started being produced by the cells, they could essentially “freeze” the pattern of HOX expression, and engineer neural stem cells that have specific HOX profiles. They could then take these neural stem cells and differentiate them further into, for example, motor neurons, that maintain the characteristics of neurons that localize to the corresponding region of the hindbrain or spinal cord.
Ashton is now collaborating with the Institute for Clinical and Translational Research at the University of Wisconsin-Madison to conduct transplantation experiments and test whether matching derived neural tissues with the location of their transplantation in the hindbrain or spinal cord helps the tissues to engraft better and therefore have a stronger regenerative effect.
–Adityarup Chakravorty